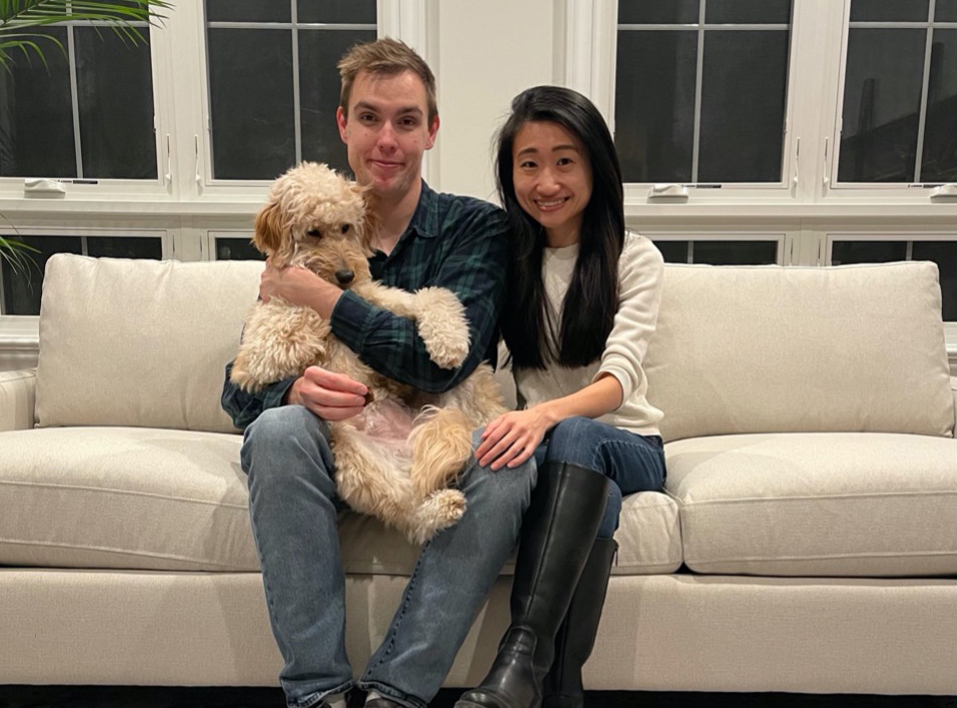
Chris Tonge and Chanel La with their dog Chuckles in their Chicago home. (Courtesy of Chanel La)
Chris Tonge and Chanel La with their dog Chuckles in their Chicago home. (Courtesy of Chanel La)
By Brittany Edelmann
Chanel La sat at the front of the nanomaterials class ready for the first day of her Ph.D. studies six years ago. She brought four pencils, several pens and a water bottle for exam day. She read her answers over and over again.
Chris Tonge sat all the way in the back of the classroom and seemed more “chill.” He showed up to class with one pencil for an exam and was one of the first to finish, La said.
They both were attending The University of British Columbia pursuing doctorate degrees in chemistry. Then one night, La needed to get into the nuclear magnetic resonance room, a lab with a giant magnet that allows for visualization of molecular structure. There’s only one room like it that all the graduate students shared and La needed to drop off her sample. La forgot her keys in her lab so she texted the group chat seeking help from another first-year student.
She asked if anybody could let her into this room. Everyone responded they were already home, since it was 10 o’clock, except Tonge. So, Tonge let her in and they had their first real conversation.
“I was surprised that he worked so hard,” La said, considering he was the only one who was still working in the lab. After that conversation, they planned to go on a date to the aquarium and to get ramen.
“The rest is history,” Tonge said.
La’s mom is from Malaysia and her dad is from Vietnam. Tonge’s dad is from England and his mom is from Spain. La sat in the front of the class and Tonge in the back.
But the chemistry is there. “We just mesh really well,” Tonge said.
And chemistry is taking their research in innovative directions. During their Ph.D. program, they both focused on making polymers, organic compounds with a sequence of units repeated. La focused on protons as a building block. And Tonge? Electrons. A proton is a positively charged subatomic particle and an electron is negatively charged. It’s as if their differences and the natural pull of negative charge to positive charge drew them together.
They also made discoveries within their own research during their studies. La created a library of drug molecules and one of them is promising for helping dissolve blood clots without the problematic side effect of promoting bleeding. Tonge helped create experimental emitters for OLEDs, or organic light-emitting diodes, that could make televisions more efficient with capabilities to last longer, save energy and create purer color.
La’s high-school science teacher piqued her interest in chemistry, which led her to continue studying chemistry in graduate school and focus on molecules to help decrease blood clots.
Depending on where they move in the bloodstream, blood clots can lead to strokes, heart attacks and breathing problems. Surgery and several physical conditions increase the risk of blood clots from forming. While current antithrombotic agents are available such as heparin, they can increase the risk of bleeding, said Jayachandran Kizhakkedathu, La’s professor at UBC and principal investigator of the research.
The goal of La’s research was to create a drug molecule that can prevent thrombosis, or clotting of the blood inside a blood vessel, without the side effects of bleeding.
To reach this goal, La worked with experts who focused on enzymes and proteins within the clotting cascade. The clotting cascade is “the series of enzymatic events that occur for a blood clot to form,” La said.
Previous research from James Morrissey, a biological chemistry professor at the University of Michigan, discovered that polyphosphate, a naturally occurring, negatively charged biopolymer, plays multiple roles within the clotting cascade, and primarily speeds up the clotting process.
La explains how many current anticoagulants target key factors within the clotting cascade such as thrombin. Other drugs can inhibit different key enzymes within the clotting cascade, Kizhakkedathu said. La was looking to design polymers that inhibit and target polyphosphate.
“If you can inhibit polyphosphate, you’re not inhibiting one of the key factors like thrombin, and you may have reduced bleeding as a side effect,” La said.
Previous studies show positive polymers like polyethyleneimine do bind to polyphosphate. However, because these polymers are extremely positively charged, they not only bind to polyphosphate but other essential negative polymers in the body. This can be toxic and ultimately lead to other side effects.
So, La designed and synthesized a library of polymers. The core of the polymer is globular and she functionalized it with “positive partners that would bind to the polyphosphate.”
The goal was to have enough positive charge on the molecule that it can bind to polyphosphate without binding to the other essential negative ions within the blood that can lead to toxic side effects.
“You’re trying to find that balance. Positive enough, but not too positive,” La said.
The next step was to conduct research with in vitro studies, studies within the lab, and in vivo studies, or studies using living animals. Three of the drug molecules did well in vitro and moved on to in vivo studies with mice.
La traveled to Michigan where she worked with Morrissey to look at the drug molecule more closely. She looked at whether the drug by itself would induce bleeding by administering the drug to a mouse and letting it circulate for five minutes. Then she cut the tail and measured the bleeding time and quantity of blood loss. This is compared to a control using saline and heparin.
Based on this work, La’s university and University of Michigan have since applied for a provisional patent on these set of drug molecules and researchers are studying the effects in large animal models. After getting the patent, the next steps are to build a body of evidence to show the efficacy and safety of the drug, La said. Then the drug molecule will hopefully be tested with humans.
“The holy grail for thrombosis treatment is to develop a drug molecule which could prevent thrombosis, but also won’t have any side effects such as bleeding,” Kizhakkedathu said. “We are close to that, but we don’t know,” considering more evaluation needs to be done on larger animals and then humans. This process can take years.
“The basis of the technology is to adapt beyond polyphosphate and is a valuable platform to design more positive polymer therapeutics,” La said.
Tonge’s love for chemistry started when he was in middle school. He loved doing experiments where significant visual changes were observed, such as foaming and color changes. This love of chemistry led him to pursue a Ph.D. in organic chemistry, where he focused on developing and producing emissive compounds of many different colors.
Besides making multicolored fluorescent compounds, he also worked closely developing “complex polymeric structures” using these emitters to simulate multilayer organic electronic devices such as an organic solar cell or an organic LED.
A typical LED television can be simplified to two key layers. One layer is a light emitting layer that functions as a backlight and the other layer is a color filter, which changes the color of the light to give you a pixel. Basically, these LED televisions have a part that makes “white” light across the color spectrum and another that selectively removes or tunes the color from that light to leave the color you see, Tonge said.
On the other hand, an organic LED, or OLED, makes light by specifically designing fluorescent emitters to address the colors that are being emitted by the screen. This creates very vibrant colors as well as deep black colors that are desirable to consumers. These emitters generate light by generating an excited state on an emitter using electricity followed by quenching of that excited state to emit light.
With these OLEDS, manufacturers design panels with very small, emissive pixels, the smallest subunit of the panel that “can make all the colors that you’re trying to display.” When electricity enters a pixel, it glows a specific color based on the emissive properties of the dyes used and the specific energy levels of the emitters to create the image seen on the screen. So, when you want the pixel to be red, just the red lights up. This also allows for very deep black colors because when it’s black, it just turns off. This is different than a normal televisions where the light is still on behind the color filter, leading to a slight glow even when the pixel is turned off, Tonge said.
The experimental emitters he made throughout his doctorate started off with simple electron transport and hole transport polymers for emissive devices. When working with these compounds, he found several highly emissive donor-acceptor intermediates that drew his interest. These emissive compounds emit light as electrons fall “from an excited state to a non-excited state,” Tonge said. “The color that’s emitted is defined by the energy gap from the excited state to the ground state.”
To generate high energy blue light, a high energy gap from excited state to ground state is needed. Tonge explained it’s harder to make blue pixels that last for a long time because it’s a very high energy emission. The high energy excited states required are unstable leading to emitters decomposing. When it comes to red light, it’s a “very small energy drop,” so small that the excited states can quench without emitting light. “Sometimes, instead of emitting light, your excited electron just dissipates as heat,” Tonge said. The easiest color to make with high efficiency is green as these emitters are less likely to decompose because of high energy states.
One of the highlights of Tonge’s work included designing and synthesizing a super high-efficiency red emitter. What Tonge was doing in the lab was “proof-of-concept work and not focused on making something commercially viable.” The emitter is more stable, more efficient and has higher color purity than many emitters on the market. Despite these advantages, the low-yielding synthesis makes the cost of this emitter to be very high, about “$10,000 a gram, and they need about a gram per TV,” Tonge said.
“When things break down inside an OLED, it’s because of undesired side reactivity happening that was not anticipated,” Tonge said. This usually means something in a high energy state reacts with something else instead of emitting light or even undergoes a spontaneous rearrangement that results in decomposition of the emitters. Tonge’s contributions focused on developing emitters were more “rigid” and less likely to undergo side reactions. He did this by bridging adjacent groups and by blocking the positions of the molecules where side reactions happen most frequently.
Besides simple emitters, he also worked on some more complex polymers, specifically focusing on large macromolecules called a bottlebrush polymer. Picture a pipe cleaner where there is a wire in the middle and many hairs or brushes perpendicular to that wire along its length. For his project he made a bottlebrush polymer by connecting norbornene, or a specific “handle people use to build polymers, building blocks to generate the wire in the middle of the brush. These norbornenes were modified with short, polymeric semiconductors to simulate a multilayer electronic device in a single polymer chain. In this way, he would attach a series of polymers tuned to glow specific colors or have specific properties.
“Like a tree of Christmas tree lights,” Tonge said.
He gives this example of an application of bottlebrush polymers. He was able to demonstrate that if the “brush” side arms of these polymers were segregated by electronic properties, it was possible to directly allow or prevent charge transfer between adjacent arms in the solid state. This can be observed by choosing two polymers that emit one color when they are prepared in a film, but form a complex when mixed together in a film that emits a different color. By making bottlebrushes that are separated into two distinct regions, it prevents the two polymers from mixing. Simply put, this means a mixed film can be forced to glow as if the polymers were not mixed at all.
The ability to control how components of organic semiconductors mix in the solid state is important for designing devices with long life spans and consistent performance over the life of the device.
The benefits of Tonge’s emitters include longer lifespans for the device, higher efficiencies and improved color purity. His creations aren’t used commercially, but the lab continues to explore the use of these emitters and polymer architectures for additional applications.
While certain devices may not last forever, the future for La and Tonge looks bright. They live together with their dog Chuckles in Chicago and their bond continues to get stronger with each year that passes.
The next step? Picking out a ring to solidify their bond and the magnetic force that drew them together.